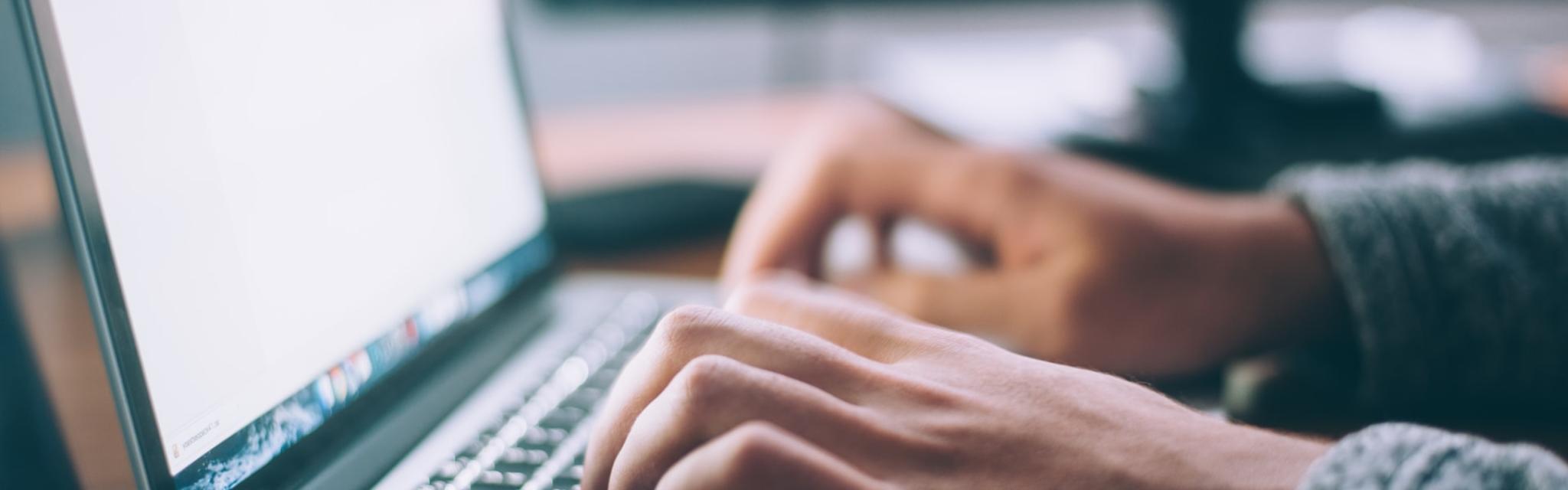
Medical implants are artificial substitutes for body parts, and materials brought into the body for functional, cosmetic, or therapeutic purposes, either permanently or removed once they are no longer needed. Prostheses can be either functional (artificial arms and legs) or aesthetic/cosmetic (artificial eye). Implants, however, tend to be used therapeutically (to heal) and are made of metal, plastic, ceramic, and even body tissues (skin, bone, etc.).
Although the implant industry has recently developed quickly, there are still no ideal devices to replace or repair bones damaged by trauma and diseases. Moreover, the human body is a very corrosive medium for implanted materials and no ideal biomaterial was invented yet to eliminate the processes occurring at the interaction with the living organism.
To readily fulfill their mission (maintain, assist or restore a person’s mobility) implants have to fit as much as possible to the patient’s unique body and be quickly tolerated.
Additive manufacturing solves clear and persistent problems in orthopedics
For the production of medical devices, doctors and patients are reliant upon custom-made designs or individualized small series. The high costs of metallic biocompatible materials and their processing increase total expenses. Cobalt alloys are difficult to machine as shape complexity rises. Therefore some implants are obtained by casting or traditional metal powder processing methods, which require tooling and is costly for small or unique series. As titanium is relatively difficult to cast in complicated shapes, titanium ingots are machined.
Recently, medical equipment manufacturers are increasingly adopting metal additive manufacturing technologies (direct metal laser sintering and electron beam melting) into the design and manufacturing of medical implants. The early use of this advanced manufacturing route for patient specific implants is encouraging. In conjunction with surgeons, engineers can produce more advanced and anatomical conforming implants and prosthesis, exploiting the power of medical imaging (X-ray, MRI, CT scans, etc…). They can build medical implants in nearly any imaginable geometry and sometimes within 24 hours.
Rapid manufacturing of custom implant is a highly valuable ability where standard implants are often insufficient for some patients facing complex cases. Previously, surgeons had to perform bone graft surgeries, use scalpels or drill implants in metal and plastic to obtain a desired shape, size, and fit.
Advantages of additive manufacturing for medical applications are:
- Individualization: Customized prosthesis/implants involve a shorter and less painful and stressful adaptation phase for the patient. Best-fit devices also make the surgical operation less difficult and stressful for the surgeon.
- Complex geometries: Some free-form structures are hardly or not feasible with conventional manufacturing methods (milling, turning or casting, etc.) while there is a growing desire to mimic bionic principles of complexity in order to accelerate the patient’s healing process.
- Functional integration: Additively manufactured medical devices are able to fulfill multiple functions with fewer components and manufacturing steps. Post-built, they can feature both a porous structure and a rough surface, improving bone integration. No further additional spray-on coating or surface texturing is required.
- Reduced surgical costs: When a patient is cared for with an efficient product, financial outlays for the hospital stay and follow-on treatment are reduced. As AM productivity increases and in-hospital process of 3D-scan-to-part speeds up, finely customized devices will enable ambulatory surgery to suffice so that patients are not kept in the hospital.
- Rapid availability: The lead time is shortened and 3D-printed medical devices can be applied sooner to patients, which is better.
Bone, the ultimate biomaterial and a source of inspiration
Fractures are doubtlessly a disastrous health issue, but bone, as a living tissue, almost instantly begins a healing process. Bone is light and strong, adapts its structure to the functional demands and self-repairs. As so, it is often perceived as the ultimate biomaterial.
Two major kinds of osseous tissues exist: trabecular (cancellous) and cortical (compact).
Major kinds of osseous tissues.
Cortical bone forms the outer shell (cortex) of most bones and accounts for about 80% of the weight of a human skeleton. It is dense and thus, relatively hard, strong and stiff.
Compared to cortical bone, trabecular bone is less dense. It has a higher surface area to mass ratio and is thus softer, weaker and more flexible. Trabecular bone is typically found at the ends of long bones, proximal to joints and within the interior of vertebrae. The greater surface area makes trabecular bone highly vascular and suitable for metabolic activity. It frequently contains red bone marrow where the production of blood cells occurs.
Plastic is often used for implants even though it is the worst material for bone formation because bone doesn’t attach to it. Most of the other implants on the market are made of bulk metal, thus are stiff and don’t deform.
The design freedom of additive manufacturing allows redistributing the material through a truss structure, it’s possible to get a significant strain with a strong metal such as titanium. Bone attach to titanium. If the titanium structure is elastic enough to deform significantly, attached cells deform with it, which triggers an osteogenic response, meaning bone formation.
Main segments in the market of orthopedic devices
The market of medical implants is an ever growing field of applications and innovation. It is segmented into 3 main classes of implants: joints, spinal and trauma.
Joint replacement remains the largest segment
Arthroplasty is a surgical procedure to restore the integrity and function of a joint, during which an artificial joint may be used. The market for reconstructive joint replacements is gaining upward momentum from the rising number of persons suffering from injured or degenerative joints. One of the causes is the aging population.
Knees and hips are highly vulnerable to degeneration caused by arthritic conditions. They account for the vast majority of reconstructive joint replacements. Other reconstructive joint replacements are shoulder systems, in a strong demand due to the advantages of orthopedic surgery over alternative therapies to reduce pain and restore arm mobility.
Advances in materials, design and processing keep improving the safety and performance of knee, hip and shoulder implants and expand their use in the treatment of severe arthritis.
Spinal implants are growing fast
A strong demand for spinal implants is created by the increasing prevalence of chronic back conditions – due to long hours spent sitting at office, for example - and a better acceptation of motion preservation surgeries as the most relevant alternative over drug and physical therapies.
This trend is fed by an availability of high value-added devices such as fusion cages, artificial discs and improved procedures for the repair of vertebrae, spinal cord injuries or abnormalities and the replacement of degenerative discs.
Trauma implants
The global orthopedic trauma market promises some growth due to increased fracture cases. More people practicing sports and the aging population result in a higher demand for orthopedic trauma internal and external fixation devices. Plate and screw systems are the most-used internal fixators for fixing a traumatized fracture and represent a sector of promising growth.
Benefits of metal additive manufacturing for major types of implants?
Hip joint replacements: AM helps reducing aseptic loosening to delay revision surgery
When the hip joint doesn’t function, due to disease or fracture, it can be replaced by a prosthetic implant either as a hemi (half) replacement or total replacement.
Today, total hip replacement is a successful and widely used surgical procedure. The concept is still based on Sir Charnley’s design and composed of a femoral prosthesis with a small diameter head, a cup and a polymer-made socket between them, to favor a low friction and smooth articulatory motion, which is critical.
Normal hip joint morphology and hip implant. The low friction polymer (HDPE) is visible within the cup.
The stem is either cemented into the femur by the means of a polymethylmetacrylate (PMM) mixture (used as a grout between the bone and the implant) or fixed cementlessly, through bone ingrowth into the implant.
Even though they need one, patients often have to wait to get an implant because surgeons know it doesn’t last for a lifetime and revision surgeries are often more complicated than the initial orthopedic surgery. Most people can only go through 1-3 revision surgeries. Improving hip implant's durability is crucial for patients. Mechanical fatigue failure is rare and the most common reason for revision surgery is “asceptic loosening”, which usually occurs 10 to 20 years after implantation and is caused by mechanical and biological factors.
An important biological factor is the biological response to wear debris, primarily generated from the prosthetic joint articular surface. The sustained chronic inflammatory response initiated at the implant-bone interface is manifested by recruitment of various cell types including most importantly osteoclasts, the principal bone resorbing cells. Signs and symptoms of the subtle progression of tissue destruction around the implant may not be clinically apparent until late stages of failure, which is insidious. Reducing as much as possible the friction coefficient of the material at the joint helps reduce biological factors.
Mechanical factors include implant-bone “micro-motions” and “stress shielding”. Micro-motions result from an inadequate initial fixation leading to a mechanical loss of fixation over time. Stress shielding is caused by the unnaturally high stiffness of the implant compared to the bone material. The stiff implant bears too large a share of the load and the surrounding living bone tissue adapts to the low stress level and resorbs.
Additive manufacturing offers a way to counteract aseptic loosening due to mechanical factors. In addition to the achievement of a better stability during the initial orthopedic surgery with patient-specific geometry, complex surface structures such as open cellular structures (for example acetabular cups that integrate trabecular structure) are designed for good bone ingrowth and improved flexibility to reduce or eliminate stress shielding.
Knee joint replacement: Patient-specific systems allow perfect fit and higher load bearing capacity
In the knee are found the most complicated joints of our body. It is composed of two joints between the leg’s bones.
- The prominent joint is located between femur and tibia while the smaller one is between the kneecap (patella) and the femur.
- A smooth and tough articular cartilage that covers the ends of the bones and facilitates sliding over each other.
- A lubricating synovial fluid, produced by the synovial membrane over the other surfaces of the knee joint to reduce friction.
Anatomy of the human knee.
Many factors can aggravate the wear rate of the bearing surface and increase the likelihood of osteolysis (active resorption of bone matrix). Femoral and tibial components loosening, torn ligaments and mobile bearing components are examples.
Indeed, the flexibility and articulation of the joint is jeopardized if articular cartilage is damaged or worn to the extent that the ends of the bones rub or grind against each other. Ultimately, it will lead to severe pain, swelling and stiffness. To alleviate the effects of severe knee damage, the patient may be oriented to the knee replacement surgery during which damaged cartilage and bone from the surface of the knee joint are removed and replaced with artificial surfaces.
In the long run, an implant’s stability depends on how well bone grows into it (screws can be used to stabilize the implant too).
Conventional knee systems are standardized and approximated to patients’ knees, based on anatomy data collected in a specific region. A standard model used to be selected first by surgeons, based on a patient’s knee shape and size, and necessary adjustments were performed during the surgical procedure.
Illustration of a traditional knee implant.
The typical causes of complications associated with traditional knee systems are:
- An uneven stress distribution in the femur that may lead to loosening of the joint
- The use of an imperfect size of tibial implant when the surgeon is unable to find a perfect one in the product portfolio. An oversized implant might damage the soft tissues and ligaments around the knee joint while an undersized implant might protrude into the cancellous bone. In both case, post-surgical pain can be expected.
- The Ultra High Molecular-Weight Polyethylene (UHMWPE) placed over the tibial tray can wear-out. Wear particles of UHMWPE induce osteolysis, a lack of mobility and pain. Eventually, a revision surgery is required. UHMWPE serves to avoid metal-to-metal contact between the femoral and tibial implants but this problem occurs if there is unintended micro motion between the tibial tray and the UHMWPE. In the worst cases, the bearing surface completely wears out until the metal-to-metal contact occurs.
- The insertion of the femoral implant might require resurfacing the patella to fit, which in many cases causes post-operative pain.
Beside this, traditional manufacturing methods like CNC machining, investment casting or plastic molding have a long lead time because they need specific tool’s design, development and production.
In recent times, the appeal of personalized knee systems has increased among surgeons and clinicians because they are designed on a principle that factors in the patient’s unique anatomy and lifestyle.
With a just in-time response to a surgeon’s request, metal additive manufacturing is a technique that allows the fabrication of personalized knee system that exhibit an almost perfect fit, uniform stress distribution and thus a higher load bearing capacity.
The better alignment allows the surgeon to perform cuts in the bone and soft tissue exactly where they need to be which reduces the risk of fat embolism and intraoperative bleeding due to minimal bone removal. There is also less tissue loss, a shorter recovery time and less postoperative pain or infection.
Finally, less instrumentation is needed and fewer efforts are required for the orthopedic surgery to position adequately 3d-printed implants.
Spinal implants: A superior design empowered by additive manufacturing
Anterior cervical discectomy and fusion (or ACDF) surgery serves to remove herniated or degenerative spinal discs. A discectomy literally "cuts out the disc" anywhere along the spine from the neck to the low back. Along this procedure, different devices can be inserted to restore patient's motion:
Interbody fusion cage
“Anterior cervical discectomy” has matured into a safe and effective procedure for the treatment of degenerative disc disease. In this procedure a hollow implant can be inserted in the spine to restore physiological disc height, allowing bone growth within and around them to stimulate bone fusion. Interbody fusion cages (or spine cages) have a load-sharing function and stabilize the spine. Traditionally made of PEEK, they are normally used in conjunction with titanium-made screws in patients suffering from spinal instability stenosis or others spinal degenerations.
Metal additive manufacturing improves usual fusion cages by compining the biocompatibility of titanium with the flexibility of plastic PEEK. The porous titanium cage fabricated by SLM could achieve fast bone ingrowth and better osseointegration with a superior mechanical stability than the conventional PEEK cage.
The particular density distributions give to titanium parts the same flexibility as traditional PEEK products, which is customised to be embedded in the spine. The higher strength of titanium allows the integration of inspection windows in the sidewalls of the 3d-printed implants. Thus, bone growth can be evaluated through medical imaging. The geometric freedom and functional integration of additive manufacturing offer a multitude of new options in spinal column reconstruction.
Customised disc prostheses
Instead of a bone graft or fusion cage, an artificial disc device may be inserted into the empty disc space. In select patients, it may be beneficial to preserve motion. It acts as a vertebral spacer to strengthen the spine and can be designed to fit perfectly into the vertebral plate. Both design and spacing dimensions can be adjusted precisely to the patient's anatomy in accordance with the surgeon's specifications. With laser-melting, these 3d-printed implants can be manufactured in a "one-shot" process, a solution that gets rid of downstream assembly processes.
Trauma implants, a plethora of one-off challenges
Trauma implants are intended for the surgical treatment of fractures, deformities, and tumor diseases of bones, such as those of the arms, legs, shoulders, or skull.
Cranial implants
Requirements placed on the manufacture of cranial implants are stringent. It’s really hard to standardize them because skulls have unique and irregular shapes. Naturally cranial implants need to be biocompatible, fit precisely and induce bone ingrowth into the edges of the 3d-printed implants. They also have to be permeable to allow brain fluid to pass through while minimal heat is being conducted to the cerebral tissue (especially in a sunny climate).
Additive manufacturing has been used to produce 3d-printed implants designed for individual patients in craniomaxillofacial surgery, with optimal size, shape and mechanical properties.
Internal fixation devices
Plates are devices fastened to a fractured bone to provide fixation. Bone anatomy is extremely complex in some areas. The plate may have to be bent in two directions and twisted. Traditionnaly, surgery time is needed to shape the plate until it fits the fracture as much as possible.
For surgeons, additive manufacturing provides a way to improve patient’s healing, by producing plates, with traumaspecific geometry, within the time limit associated with fractures.
Shaping the plate to the exact geometry and digitally pre-planning the screws will provide a better attachment of plates and a good foundation to accomplish low invasive surgery, which gives small scars, a shorter open-wound time and shorter recovery time.
Internal plates and fracture: Geometry is necessarily complex.
The concept of orthopedic implants obtained from metal additive manufacturing demonstrates a great potential for clinical applications. Better bone cell ingrowth and vascularization provide the basis for new orthopaedic innovations promoting better reliability and 3d-printed implants-body compatibility. As it changes the traditional paradigm, a new set of design, manufacturing and regulatory challenges have to be addressed to encourage further penetration in medical applications.
At Spartacus3D, we are convinced that medical devices manufacturers should leverage the capabilities of metal additive manufacturing to remain competitive and provide surgeons and hospital best ways to maintain, assist or restore people’s mobility. AM technologies can drive better outcomes for patients as well as healthcare providers.
To supply a growing demand, we have fully dedicated an EOS machine to the one-off production of medical grade titanium parts. We aim at providing our customers from the healthcare sector with both expertise and industrial know-how and we supply them with high-quality implants for orthopedics, spine and trauma.